Why Animal Free FAQ
Why Animal Free FAQ
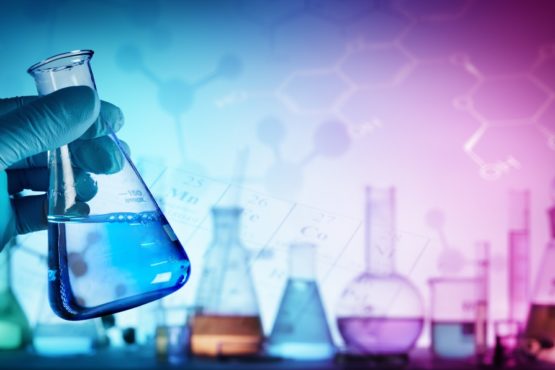
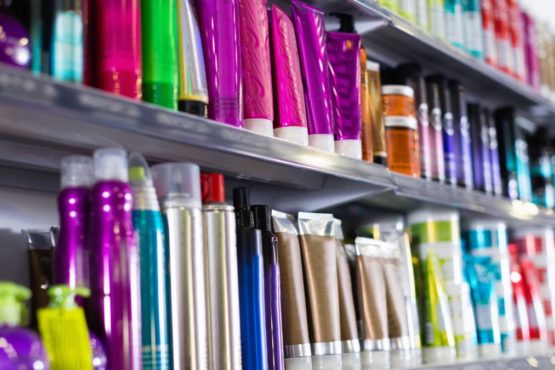
Each of us is exposed to thousands of chemicals every day – in the personal care products we use, the medicines we take, the food and beverages we consume, even the air we breathe. What most of us aren’t aware of is what goes on behind-the-scenes to assess the safety of chemicals and products, and the many laws and regulations around the world that govern this process.
Safety assessors in companies and government health authorities want to know what level of exposure will produce a toxic effect, and identify the highest exposure level that results in no harmful effect. They use this information to calculate the overall risk a substance or product poses based on how we use it or come across it in daily life. The risk of harm will depend on the type of chemical, the amount of chemical, the route of exposure (whether it was inhaled, eaten, or absorbed through the skin), and the number of times or length of exposure. Different people will have different susceptibilities depending on their age, gender, genetics, if they have any medical conditions, or if they are pregnant.
Product safety laws and regulations vary tremendously by country and product type. In some cases, animal testing may be entirely avoidable, while in others, numerous different animal tests may be expressly required before a new chemical, pesticide or medicinal product is authorized for sale.
In toxicity tests, animals are (force-)fed, forced to inhale, injected with, or have chemicals applied to their skin or eyes. A test can go on for as little as a week to as long as two years. The number of animals used in a single test can range from fewer than 10 to more than 2,000 individuals.
Safety assessors are on the lookout for signs of harm – from irritation to internal organ damage, reduced fertility or birth defects, tumors, and even death. Pain relief is withheld on the basis that it could interact with the substance being tested and contaminate the study data. Assessors then use the results from these laboratory tests on animals to try to predict real-world effects on people and/or wildlife.
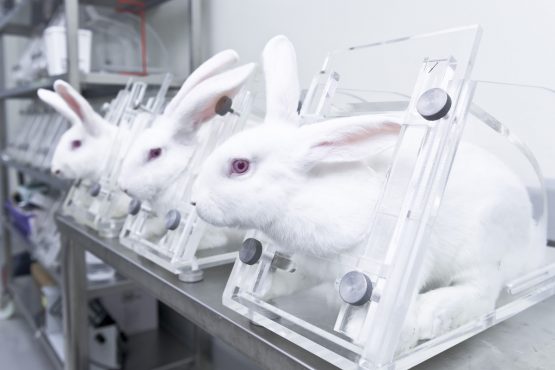
Eye irritation
In the in vivo Draize rabbit eye test, regulatory reference method, chemicals are applied to rabbit’s eyes, and changes are monitored over time. If no eye damage is observed, the chemical is not categorised. Otherwise, the classification depends on the severity and reversibility of the eye damage.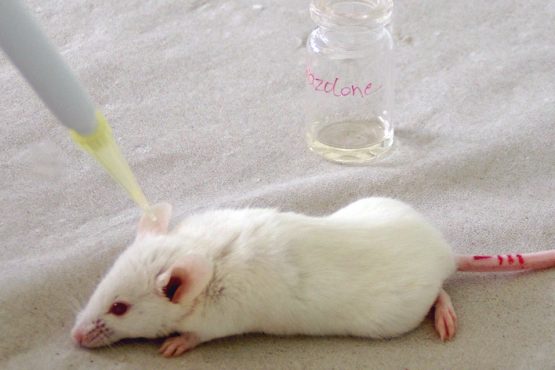
Skin allergy
Skin sensitization, resulting in allergic contact dermatitis, has traditionally relied on animal testing, such as the local lymph node assay (LLNA) in mice, and the guinea pig maximization test (GPMT). Compared with the GPMT, LLNA uses fewer animals, and has largely superseded the GPMT. It is based on measurement of proliferative responses by draining lymph node cells induced following topical exposure of mice to test chemicals.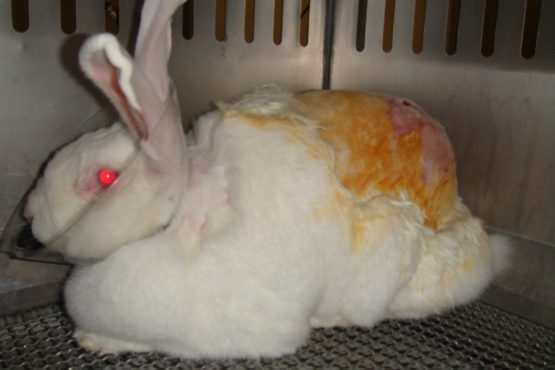
Skin irritation & dermal systemic toxicity
Dermal irritation, in terms of “reversible damage of the skin following the application of a test substance for up to 4 hours”, is generally assessed by the potential of a certain substance to cause erythema/eschar and/or oedema after a single topical application on rabbit skin and based on the Draize score.
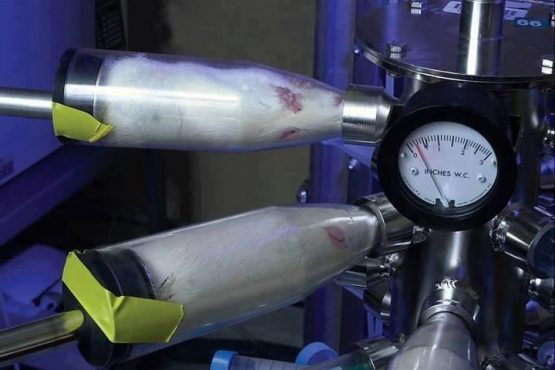
Inhalation toxicity
Subacute (28-day) and subchronic (90-day) inhalation toxicity tests rely on rodents (preferably, rats), which are nose-only or whole-body exposed to the test substance using dynamic inhalation chambers, and sacrificed at the end of the exposure period.
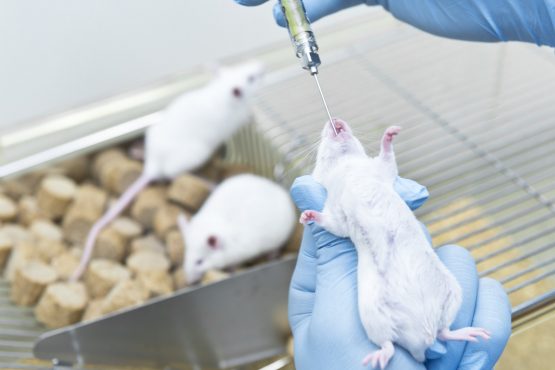
Mammalian oral systemic – rodent
Acute (14-day), subacute (28-day) and subchronic (90-day) oral toxicity tests rely on rodents (preferably, rats). Test chemical is administered by gavage, or via the diet or drinking water. All animals are sacrificed and necropsied after the dosing period.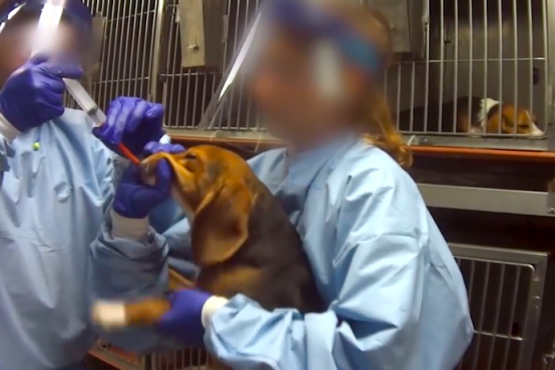
Mammalian oral systemic – dog
When effects observed in rodent studies require further clarification/characterization in a second species, or when non-rodent species are deemed to be the most relevant choice based on toxicokinetic studies, dogs are the commonly used second species. Likewise, dogs are treated with orally administered chemicals and, at the end of the dosing period, are sacrificed and necropsied.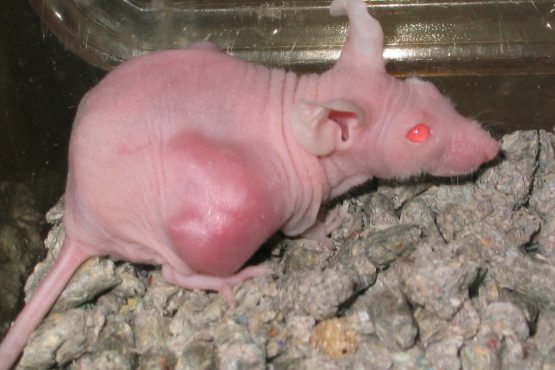
Cancer & genetic toxicity
In general, in vivo genotoxicity tests are performed following positive in vitro results, and they aim to detect DNA strand breaks, and damages induced by chemicals to the chromosomes. The classical in vivo tests are conducted with tissues, such as bone marrow, peripheral blood cells, and hepatocytes of animals, using rodents. Bone marrow cells are usually obtained from the femurs or tibias of the animals immediately following euthanasia. Similarly, other selected tissues are removed from animals, after being euthanized.
Carcinogenicity studies are usually carried out in rodent species, where the test chemical is administered daily during the majority of their life span. In fact, these tests normally last for 24 months, at the end of which animals are sacrificed and necropsied.
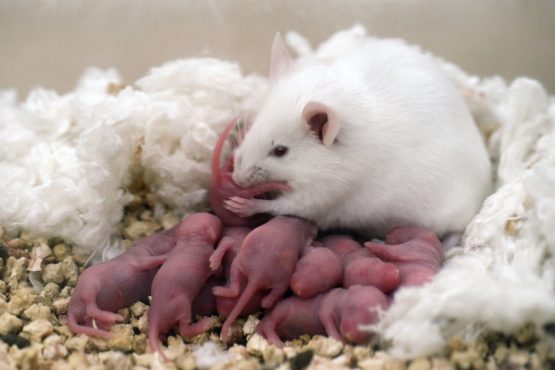
Toxicity to development & reproduction – rodent
Assessment of developmental and reproductive toxicity (DART) of chemicals is typically carried out using rodent species. Pregnant females are exposed throughout pregnancy, and multi-generational tests also involve exposing the pups throughout their lives and sometimes into the next (second) generation. In some tests, the mothers (typically a minimum of 100 pregnant animals) and fetuses (generally around 800 animals) are killed near the end of gestation and the fetuses are examined for deformities. Whichever method is used, at the end of the test, all of the animals are then killed and examined for adverse effects, with special attention paid to the reproductive systems and the health, growth, development, and function of the offspring.
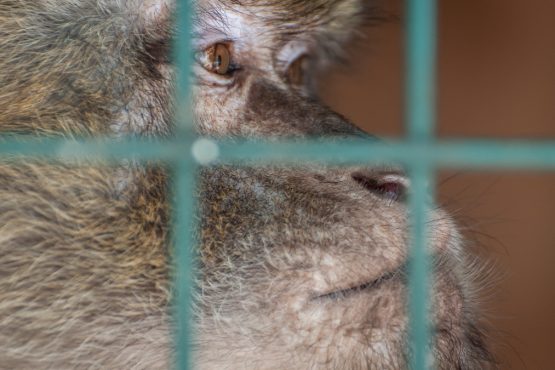
Toxicity to development & reproduction – primate
Non-human primates (are most frequently used for DART testing of biopharmaceuticals (e.g., monoclonal antibodies), when commonly used rodents are not considered as pharmacologically or biologically relevant species. Cynomolgus macaques is the most frequently used NHP. Females are exposed to a biopharmaceutical during pregnancy and lactation, in order to evaluate potential exposure and risks to embryos, fetuses and infants.
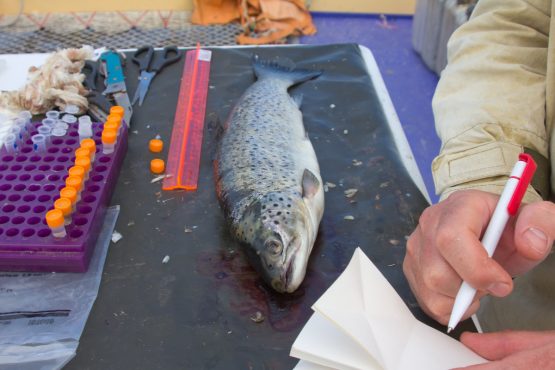
Toxicity to fish
Freshwater and marine fish species are tested in acute and chronic toxicity assays to derive protective water criteria and thresholds. These tests can last from 96-h (acute toxicity tests) to longer periods (chronic toxicity tests), covering full early-life stages, sexual differentiation, or eventually exposure over multiple generations. Generally, the recorded endpoints are mortality, growth (e.g., length, weight), time to hatch, hatching success, and any morphological, or behavioural abnormalities.
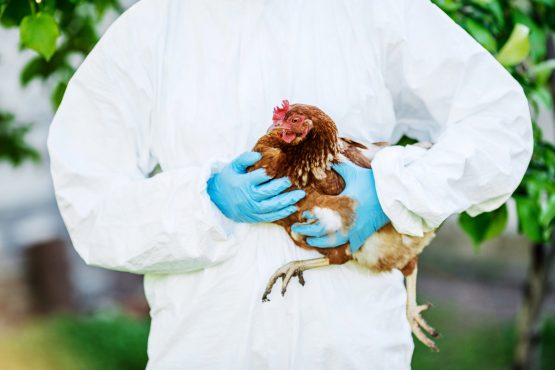
Toxicity to birds
Avian dietary (8 days) and reproduction (> 20 weeks) tests are used as acute and chronic toxicity tests with birds, respectively. In the dietary tests, the measured endpoints are mortality, signs of toxicity and other abnormal behaviour, body weights, food consumption. Whereas, endpoints such as mortality of adults, egg production, cracked eggs, egg shell thickness, viability, hatchability and effects on young birds are observed during the reproduction toxicity study. In terms of animal numbers, long-term avian studies are the most animal intensive.
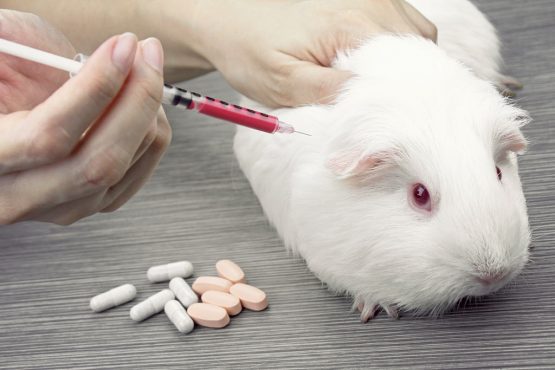
Vaccine safety & potency
In the production of vaccines, animals can be used at different stages, including testing the starting material used to produce live viral vaccines; to test product intermediates, as part of in-process control; and to test of the final product prior to batch release. Usually, in those type of tests, animals are injected with different dilutions of the vaccine and observed for any adverse reactions, injected and infected with the virulent agent, or injected and bled to measure protection against the disease to demonstrate the safety and efficacy of the product.- Acute systemic toxicity tests are short-term experiments designed to determine how much of a single dose of a chemical is needed to kill an animal. For these tests, groups of animals (usually rats or mice) are exposed to different, generally quite large, amounts of the chemical – and the animals are observed for several days to see if any get sick or die. Increased amounts of chemicals are tested until there is enough information to calculate the “lethal dose.” These tests use between 5 and 30 animals for each chemical tested. The doses in these experiments are many times (sometimes thousands of times) higher than people would normally be exposed to or that would be found in the environment.
- Acute topical toxicity tests are short-term experiments designed to determine whether topical exposure causes any immediate effects. These types of tests use primarily guinea pigs, rabbits and mice. Chemicals are applied to the animal’s skin or eye and the animal is monitored for signs of redness, irritation, allergic reaction or swelling for a few days. The animal is then killed and dissected so their body tissues can be analyzed for adverse changes.
- Repeat-dose testsevaluate potential harmful effects resulting from longer-term exposure to multiple doses of a chemical. Typically, animals are exposed daily to a chemical anywhere from 28 days to 2 years. These types of test use dozens, hundreds, or even thousands of animals – usually rats or mice (and dogs for pesticides; pigs and monkeys for drugs) – for each chemical tested, depending on the type of test. Some common repeat dose tests are:
- Subchronic (28- and 90-day) general toxicity tests are commonly required for many different types of chemicals, and usually by two routes of exposure. Throughout the test, many different aspects of the animals are measured, e.g. weight loss and signs of stress such as altered breathing, self-mutilation, reduced grooming, or tremors, blood and urine chemistry. At the end of the test, the animals are killed and dissected to observe tissue or organ damage, pre-cancerous lesions or the growth of tumors. Typically, these tests use between 40 and 80 rats, mice and/or dogs and sometimes rabbits or Guinea pigs depending on the test or route of exposure. Although the exposure levels used in these tests are generally lower than in acute tests, they are still many times higher than real-world exposures. This is done in order to see results in weeks or months in the animal tests vs the months or years of exposure these tests are trying to predict.
- Cancer tests involve exposing 400 rats or mice to a chemical for most of their natural life-spans, around 2 years for rats and 18 months for mice. The animals are observed daily for the occurrence of visible tumors. At the end of the experiment, animals are killed and dissected to look for organ changes that might indicate the presence of cancer.
- Tests for effects on Development and Reproduction are done using by exposing adult mice, rats, or rabbits prior to mating, and then exposing pregnant females throughout pregnancy. Multi-generational tests also involve exposing the pups throughout their lives and sometimes into the next (second) generation. In some tests, the mothers (typically a minimum of 100 pregnant animals) and fetuses (generally around 800 animals) are killed near the end of gestation and the fetuses are examined for deformities. Whichever method is used, at the end of the test, all of the animals are then killed and examined for adverse effects, with special attention paid to the reproductive systems and the health, growth, development, and function of the offspring. Depending on the method used, between 675 and – 2,600 animals are used for each chemical tested.
- Vaccines safety and potency tests. Biologicals, including many vaccines, are medicines that are often complex in their properties, modes of action and manufacturing processes, to an extent that was not considered possible to measure the ‘active component’ in the product using chemical or physical methods. Consequently, many of the tests designed to assure safety and potency have been based on animals. Animal testing still play a critical role in the development of biological products, like vaccines, and are used at various stages of their development (early development, pre-clinical studies, clinical studies and post-licensure as part of the product release process). In the production of vaccines, animals can be used at different stages, including testing the starting material used to produce live viral vaccines; to test product intermediates, as part of in-process control; and to test of the final product prior to batch release. Usually, in those type of tests performed to prove the safety and potency of the product, animals are injected with different dilutions of the vaccine and observed for any adverse reactions, injected and infected with the virulent agent, or injected and bled to measure protection against the disease.
- Ecotoxicological studies investigate the toxic effects of substances on species in ecosystems (aquatic, terrestrial) in terms of changes in the state or dynamics at their organism level, or at other levels of biological organization. As an example, the investigation of toxic effects of chemicals on species living in water is determined with organisms representing the three trophic levels: algae or plants, invertebrates (e.g., crustaceans), and vertebrates (generally, fish). Ecotoxicological laboratory toxicity tests are usually single-species tests in which the toxicity of a chemical is measured through mortality, decreased growth rate and lowered reproductive capacity. In more detail, acute toxicity tests measure the severe effects (e.g., mortality, immobility) suffered by organisms from short-term exposure to substances, whereas chronic toxicity tests study effects over prolonged periods of exposure (e.g., the entire life cycles) and usually the endpoints are sublethal (e.g., growth) or measurements of reproductive/developmental effects. Acute toxicity data are widely required by regulatory authorities as a base set requirement, whilst chronic toxicity tests may be needed in a tiered testing requirement based on exposure levels, and/or a specific hazard concern (e.g., endocrine activity), and/or to gain more ecologically relevant data. The EU summary report, under Directive 2010/63/EU, on the statistics on the use of animals for scientific purposes by the Member States of the Union, released in early 2020, reports a total number of animals’ uses in acute, chronic, reproductive, endocrine activity, bioaccumulation, and other ecotoxicity tests of 44.915, 28.738, 1.450, 5.924, 4.110, and 9.210, respectively, for a total number of 94.347 animal uses for regulatory ecotoxicological purposes in 2017.
As an example, research for drug discovery and development has relied heavily on the use of preclinical animal models. Nevertheless, more than 90% of compounds entering clinical trials fail to gain regulatory approval, mainly as a result of insufficient efficacy and/or unacceptable toxicity in humans, due in large part to the limited predictive value of preclinical studies (Plenge et al., 2013, in Langley et al. 2017). The lack of translation from animal studies to humans has several causes, including important differences between species, the fact that animal models do not fully mimic human diseases, lack of understanding of underlying mechanisms of diseases, as well as experimental design flaws and bias (Institute of Medicine, 2014; Pound and Bracken 2014).
Overall, there is a growing recognition that, to increase the success rate, a stronger focus on human-relevant data is therefore needed (Langley et al., 2017), moving from a system that relies on animal assays to one that aims to identify and characterize specific mechanisms or pathways that lead to adverse effects in humans, to design assays to measure pathway responses, to develop models that can predict toxicity using the assay data, and to set priorities among chemicals for more comprehensive toxicity testing (NRC, 2007; NASEM, 2017).
Decades of animal testing has pointed to some limitations that newer technologies and approaches can help improve or overcome:
Animal testing is time-consuming and expensive and limits the number of chemicals and products that can be tested
For example, to test a single active ingredient in a pesticide, it takes a about a decade, costs approximately $71,000,000 and kills up to 8,000 animals to complete all of the animal studies required to register one single pesticide. And it’s not only pesticides: many countries now require that industrial chemicals –in everything from clothing and furniture to kitchen appliances and electronics – are tested, and this testing often involves the same or similar tests as are used for pesticides. It’s estimated that there are between 35,000 and 100,000 of these chemicals already in our surroundings, with thousands of new chemicals created every year. Using animal testing to evaluate the safety of all of these chemicals would take billions of dollars, decades, and hundreds of millions of animal lives.
In contrast, non-animal methods such as computer modelling can reduce the time required to evaluate a chemical from years to weeks or days, often at substantially less than the cost of animal tests.
Animals are not simply small humans
Another problem with animal testing is that it assumes humans are just giant rats, mice, rabbits, or other experimental animals. Sure, there are some key similarities in basic biology, cells, and organ systems, but there are also differences, and those differences can make a big… well… difference!
Four main factors help determine how chemical exposure will affect an animal: how the chemical is absorbed, distributed throughout the body, metabolized, and eliminated. These ADME processes can vary greatly from species to species – and this can lead to critical differences in the effects of chemical exposure between species. Researchers try to use animals that are a close match to humans in ways that are likely to matter for the chemical being tested. If they’re concerned about potential effects on the heart, for instance, they might choose a dog or pig – because the circulatory systems in these animals are more similar to humans than other animals are. If they’re concerned about the nervous system, they might use cats or monkeys. But even with a relatively good match, differences between species can make it difficult to translate animal results into human risk, especially since small differences in biology can have large effects on ADME processes.
For instance, rats, mice and rabbits have skin that absorbs chemicals quickly—much faster than the skin of humans does. So, tests using these animals can overestimate the danger of chemicals that are absorbed through the skin. Even something like how much mucus an animal produces to line its stomach can affect how much and how quickly a chemical is absorbed into the bloodstream.
As a consequence, drug discovery built on a foundation of animal testing has proven limitations: a single drug on the market comes at the cost of more than a billion dollars, more than a decade of research and countless animal lives. And, as mentioned above, a very high failure rate in clinical studies. But before the clinical trials in humans, each of those failures had looked good in numerous animal tests, usually in several different species. And everyone has heard of medicines that crash and burn after they make it into drugstores and kitchen cabinets. The arthritis medication Vioxx, the weight-loss drug fenfluramine (the “Fen” in “Fen-Phen”), and the allergy medicine Seldane were all pulled from the market after numerous reports of cardiovascular side effects, such as heart attack, stroke, arrhythmia, and heart disease. Animal tests had given these drugs an all-clear. On the flip side, how many potentially helpful drugs have been abandoned by misleading findings in animals? We’ll never know for sure, but one example would be aspirin: aspirin happens to be quite toxic to dogs and would likely never have made it to human clinical trials if it had been discovered in the latter half of the 20th century, when we adopted the animal testing paradigm for drug safety.
In contrast, non-animal methods that use human cells or incorporate human data from real-life exposures, give researchers a more accurate picture of how humans respond to a chemical.
The high exposures used in animal experiments give misleading results
When scientists study a chemical, they’re often trying to figure out if it’s safe for a person, or plants and animals in the environment, to be exposed to a very small amount of it for years at a time. After all, you don’t eat a tube of toothpaste every morning and night. Similarly, you don’t eat the chemicals used to make plastic food containers, but you care about the safety of those chemicals because small amounts may get into your leftovers. In other words, scientists are frequently trying to answer questions about the safety of long-term exposure to low levels of a substance—but it’s impossible to study these long-term effects in animals, since most of them don’t live nearly as long as humans, and toxic effects are usually rare. In order to try to see these toxic effects in a short time frame, scientists expose animals to much higher doses of chemicals than humans would ever experience or would ever be seen in the environment (animal testing normally requires that the top dose in experiments shows some signs of overdose).
In fact, these tests generally require concentrations of the chemical that are thousands of times higher than someone would experience in a typical real-world exposure. However, this approach doesn’t make problems show up thousands of times faster – it makes different problems show up. It’s a little like tossing a rat into a vat of gasoline and then concluding that gasoline causes drowning. The rat dies of other causes before tests can show the neurological damage that can be caused by long-term, low-dose exposure to gasoline. All we can possibly learn from high-dose experiments is what might happen in overdose situations. And we don’t learn what might actually happen with exposures to lower concentration for longer periods of time.
In contrast, combining different non-animal methods (such as computer simulation and human cell-based models) can be used as an efficient way to evaluate a chemical’s overall biological activity relative to other chemicals and to give toxicologists a good idea of what kind of toxicity it might induce. Additionally, monitoring real-life, daily exposure of people can help us understand what effects low level, long-term exposure has. These population-based studies have been shown to provide information on health risks that have not been revealed by animal testing.
Increasing numbers of people find animal testing unethical
The public has become less supportive of animal testing as awareness of the issue grows. The Pew Research Centre Survey from 2018 indicated that over half of the respondents in the US were opposed to the use of animals in scientific research and successive Gallup opinion polls have revealed a decrease in the view that animal testing is morally acceptable. In contrast, public support for the use and development of non-animal methods is on the increase, as illustrated by a 2016 UK opinion poll that showed that the majority of the British public wanted to see more research on non-animal methods, with growing support for a total ban on animal testing.
Legal incentives limiting animal testing
In the European Union, the overarching Directive 2010/63/EU lays down the main measures for the protection of animals used for scientific or educational purposes. Every country is implementing the bill in its own way; however, under this legislation, every procedure involving a vertebrate animal that is proposed in the EU requires approval from a federal oversight committee. The Cosmetics Regulation (EC) No. 1223/2009 bans the marketing of cosmetics products and ingredients in the EU that have been tested on an animal since 2013, irrespective of the fact that full replacement methods are not yet available for complex health effects. Several other countries and states have passed similar bans since then (see Cosmetics: Global regulatory alignment). The United Kingdom has gone a step further and outlawed testing household chemicals (e.g., cleaning and laundry products, air fresheners) on animals. And more countries are likely to adopt these bans, too, as more and more people object to chemical testing on animals.
Articles 13(1) and 25(1) of REACH Regulation (EC) No. 1907/2006 on chemicals state that testing on vertebrate animals shall be undertaken only as a last resort, and information shall be generated whenever possible through the use of alternative methods. In the U.S., the Toxic Substances Control Act (TSCA) updated in 2016 directs the Environmental Protection Agency (EPA) to reduce and to replace the use of vertebrate animal in the testing of chemicals, and to promote the development and timely incorporation of alternative test methods or strategies. In this regard, on September 10, 2019, U.S. EPA Administrator Andrew Wheeler further reiterated the commitment of the Agency to move away from animal testing, by signing a directive to prioritize efforts to reduce animal testing, concluding that: “Through scientific innovation and strategic partnerships, we can protect human health and the environment by using cutting-edge, ethically sound science in our decision-making that efficiently and cost-effectively evaluates potential effects without animal testing”.
Micro-physiological systems: 3D cell models, organoids, organs-on-chips
3D cell culture is a cell culture method that allows cells to grow in all direction in an in vitro condition. There are many variations of 3D cell cultures, which show great promise for understanding both normal human physiology and disease mechanisms (Marshall et al., 2018).
As an example, microfluidic organs-on-a-chip is an emerging 3D cell culture field that recreate the structural and functional features of human organs. Microengineered, so-called ‘microphysiological systems’ (MPS), contain cultures of human cells with dynamic fluid flow that recreate nutritional delivery, mechanical forces, and biomolecular gradients, such as those experienced in vivo, and aim to emulate biological processes at a microscopic scale (Marshall et al., 2018). So far, functions of various organs and tissues, such as the lung, liver, kidney, and gut have been reproduced as in vitro models. Furthermore, a body-on-a-chip or human-on-a-chip, integrating multi organ functions on a microfluidic device, has also been proposed for prediction of organ interactions (Kimura et al., 2018).
Organoids are a new type of 3D culture models that have emerged in recent years. They are essentially miniaturized organs generated from stem cells in vitro. Under appropriate growth factor treatments, these cells differentiate and self-organize into organ-specific cell types and tissue organization. Organoid models have the potential to complement or even substitute for animal models. Compared with animal models, the organoid model can be scaled-up for high throughput testing at a lower cost with fewer ethical concerns. In addition, it can be used to study human diseases that are difficult to be modeled accurately in animals (Yu et al., 2019).
Computer models
Nowadays, size and complexity of biological data is constantly increasing, especially due to advancements in high-throughput sequencing technologies. Computer models based on mathematical and statistical techniques are then used to build a coherent view of what all these data mean.
In the systems toxicology, advanced computational methods aim to study how networks of interacting biological components determine the properties and activities of living systems, by integrating data from all levels of complexity (genomics, proteomics, metabolomics, and other molecular mechanisms). The goal is to create overall computational models of the functioning of the cell, multicellular systems, and ultimately the organism. These in silico models will provide virtual test systems for evaluating the toxic responses of cells, tissues, and organisms.
The science of managing and analyzing biological data using advanced computing techniques is defined bioinformatics (Alpi, 2003), and it includes computational tools that mine and analyze information from large databases of biological data.
Additional computer models, already accepted at a regulatory level for some (eco)-toxicological endpoints, are the so-called QSAR (Quantitative Structure-Activity Relationship) methods, which are important for prediction of biological effect of chemical compounds based on mathematical and statistical relations.
Pathway-based toxicology
The pathway-based toxicology is a new paradigm in which biologically important perturbations in key toxicity pathways, i.e. Adverse Outcome Pathways (AOP) are evaluated with new approach methodologies in molecular biology, bioinformatics, computational toxicology, and a comprehensive array of in vitro tests based primarily on human biology. The concept of using knowledge of biological pathways or networks (systems biology/toxicology) serves different functions, the first to discover previously unknown pathways and associations, the second to form a reliable basis upon which to found hypothesis-driven research, read-across or regulatory decisions (Marshall et al., 2018). Systems toxicology is expected to enable the shift from toxicological assessment using solely apical endpoints toward a pathway-based approach to safety testing.
Alternative methods for vaccines’ safety and potency tests
While it is still considered a challenge completely replace the use of animals in the vaccines’ R&D, improved approaches (e.g. genomics, proteomics, bioinformatics, reverse vaccinology, Quality by Design) were able to define novel vaccines, registered with final safety and potency tests with less or no animals. In addition, vaccines’ production is also slowly moving out from the use of embryonated eggs or animal primacy cell lines. In the legacy vaccines (products that are on the markets since decades), some alternative methods to refine, reduce and replace in vivo potency and safety tests are accepted by some regulators and implemented by the manufacturers; and in some cases, obsolete tests in vivo tests (e.g. general safety test) have been waiver or deleted by the regulatory requirements (Lang et al., 2018). For some viral vaccines (e.g. flu vaccines) and well-defined vaccines (e.g. meningococcal polysaccharide conjugate vaccines), a potency test can be performed entirely in vitro via antigen quantification assays or tests to assure the presence of the conjugated molecule. For more-complex vaccines, testing may involve the immunization of animals where humane end-points or single dilution testing scheme could be applied in order to refine the procedure and reduce the number of animals used.